Work Ratio Definition In Thermodynamics
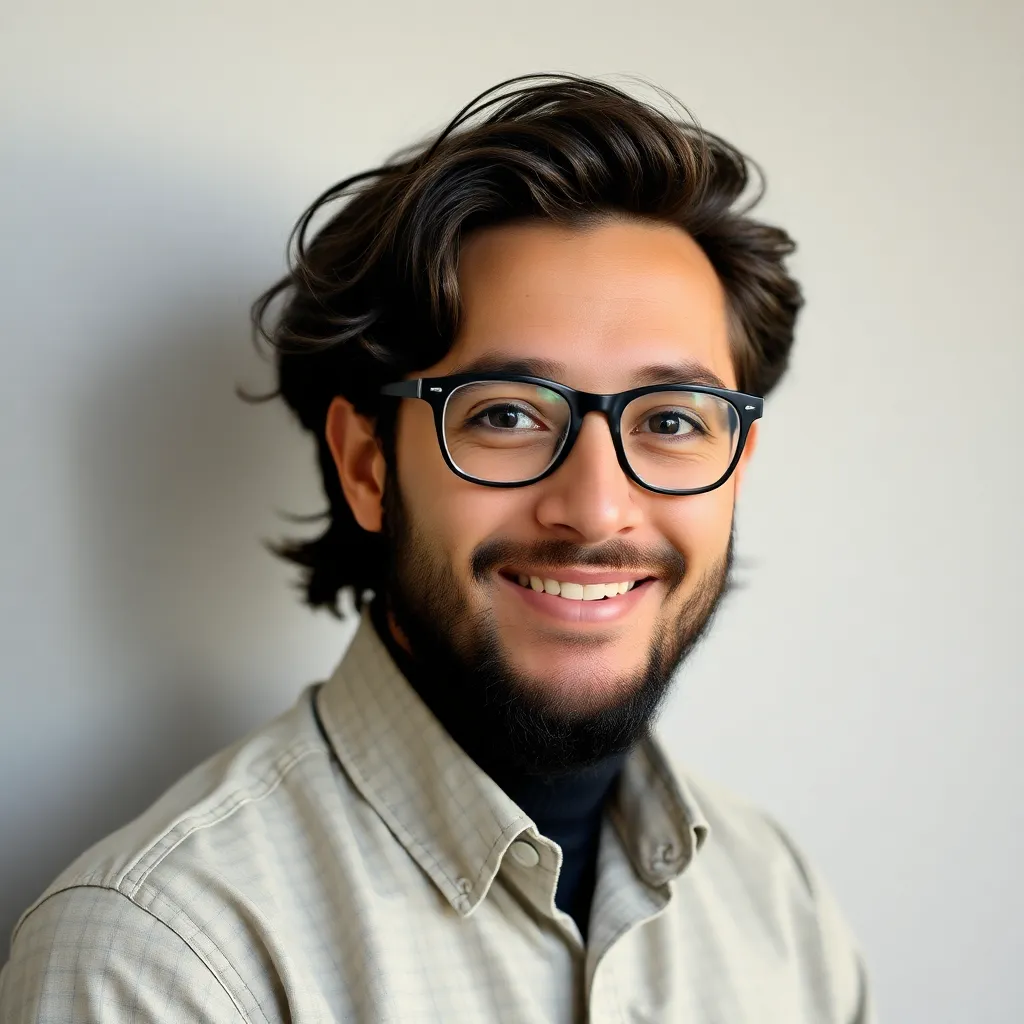
adminse
Mar 29, 2025 · 9 min read

Table of Contents
Unveiling the Mysteries of Work Ratio in Thermodynamics: Efficiency, Applications, and Beyond
What is the true significance of work ratio in thermodynamic systems, and how does it impact overall efficiency?
Work ratio, a critical parameter in thermodynamic analysis, offers profound insights into the performance and efficiency of various systems, from power plants to refrigeration cycles.
Editor’s Note: This comprehensive exploration of work ratio in thermodynamics has been published today, providing up-to-date insights and analysis for engineers, students, and anyone interested in understanding thermodynamic efficiency.
Why Work Ratio Matters
Understanding work ratio is crucial for optimizing the performance of thermodynamic systems. It provides a direct measure of how effectively a system converts input energy into useful work. This metric is relevant across various applications, including:
-
Power Generation: In power plants (e.g., steam power plants, gas turbine power plants), a higher work ratio indicates better utilization of fuel and increased power output for a given input. Analyzing work ratio allows for the optimization of the cycle's design and operating parameters.
-
Refrigeration and Air Conditioning: In refrigeration cycles, the work ratio is inversely related to the coefficient of performance (COP). A lower work ratio translates to a higher COP, meaning more cooling effect is achieved per unit of work input. This is essential for designing energy-efficient cooling systems.
-
Heat Pumps: Similar to refrigeration cycles, heat pumps rely on the work ratio to assess their efficiency in transferring heat from a lower temperature source to a higher temperature sink. Optimizing the work ratio directly impacts the heating capacity and energy consumption.
-
Internal Combustion Engines: In internal combustion engines (ICEs), the work ratio helps evaluate the engine’s efficiency in converting the chemical energy of fuel into mechanical work. It reflects factors like combustion efficiency, heat losses, and friction.
-
Process Engineering: In industrial processes involving thermodynamic cycles, the work ratio guides process optimization, allowing for minimizing energy consumption and maximizing product yield.
Overview of the Article
This article delves into the core principles of work ratio, exploring its definition, calculation, significance in different thermodynamic cycles, and the factors influencing it. Readers will gain a comprehensive understanding of this critical parameter and its role in improving the efficiency of various systems. We will also examine its limitations and explore future research directions.
Research and Effort Behind the Insights
The insights presented here are based on extensive research drawn from established thermodynamic textbooks, peer-reviewed scientific articles, and industry reports. The analysis incorporates fundamental thermodynamic principles and real-world applications, ensuring the accuracy and relevance of the information.
Key Takeaways
Key Aspect | Description |
---|---|
Definition of Work Ratio | The ratio of net work output to the total work input in a thermodynamic cycle. |
Calculation Methods | Varies depending on the specific cycle; generally involves calculating net work and work inputs/outputs. |
Significance in Efficiency | Directly reflects the system's ability to convert energy into useful work; higher ratio indicates better efficiency. |
Cycle-Specific Analysis | Work ratio interpretation differs for Carnot, Rankine, Brayton, and other cycles. |
Impact of Losses | Friction, heat losses, and other inefficiencies reduce the work ratio. |
Optimization Strategies | Designing systems to minimize losses and maximize energy conversion. |
Smooth Transition to Core Discussion
Let's now delve into the detailed explanation of work ratio, starting with its fundamental definition and moving on to its application in various thermodynamic cycles.
Exploring the Key Aspects of Work Ratio
-
Definition and Calculation: Work ratio is defined as the ratio of the net work output to the total work input in a thermodynamic cycle. Mathematically:
Work Ratio = Net Work Output / Total Work Input
The calculation of net work output and total work input depends on the specific thermodynamic cycle being analyzed. For example, in a Rankine cycle, net work output is the difference between turbine work output and pump work input. The total work input could be the heat added to the boiler or the energy input to the pump. Different cycles will require different calculations based on their specific processes.
-
Work Ratio in Different Cycles: The interpretation and significance of work ratio vary across different thermodynamic cycles.
-
Rankine Cycle (Steam Power Plants): A higher work ratio indicates better turbine efficiency and less energy consumed by the pump. Optimizing the cycle parameters (pressure, temperature, etc.) aims to maximize the work ratio.
-
Brayton Cycle (Gas Turbine Power Plants): The work ratio reflects the efficiency of the compressor and turbine. Improving the turbine's efficiency and minimizing compressor work are crucial for increasing the work ratio.
-
Carnot Cycle (Theoretical Ideal Cycle): While the Carnot cycle serves as a theoretical benchmark, its work ratio is still an important concept for understanding the limitations of real-world cycles.
-
Refrigeration Cycles: In refrigeration and heat pump cycles, the work ratio is often expressed inversely as the coefficient of performance (COP). A high COP (or low work ratio) indicates high efficiency in transferring heat.
-
-
Factors Affecting Work Ratio: Numerous factors can influence the work ratio of a thermodynamic system:
-
Internal Losses: Friction within moving parts (turbines, pumps, compressors) reduces the net work output, lowering the work ratio.
-
Heat Losses: Heat transfer to the surroundings from various components reduces the overall efficiency and lowers the work ratio.
-
Isentropic Efficiency: Deviations from ideal isentropic processes (e.g., adiabatic compression/expansion) affect the work output and, consequently, the work ratio.
-
Pressure Ratio (in Gas Turbines): The pressure ratio significantly impacts the work ratio in gas turbine cycles. Optimizing this ratio is crucial for maximizing efficiency.
-
Temperature Limits: The maximum and minimum temperatures in a cycle directly influence the work output and the work ratio.
-
-
Optimization and Improvement: Improving the work ratio involves strategies aimed at reducing losses and maximizing energy conversion. These strategies may include:
-
Improving Component Efficiencies: Using advanced materials and designs to reduce friction and enhance heat transfer.
-
Optimizing Cycle Parameters: Adjusting pressure ratios, temperatures, and other parameters to maximize net work output.
-
Minimizing Heat Losses: Implementing better insulation and heat recovery systems.
-
Advanced Control Systems: Employing sophisticated control systems to optimize the operation of the thermodynamic cycle based on real-time conditions.
-
Closing Insights
Work ratio serves as a vital indicator of efficiency in thermodynamic systems. Understanding its significance across various cycles and the factors influencing it is paramount for engineers and researchers. Optimizing the work ratio translates to substantial improvements in energy efficiency, reduced operational costs, and a smaller environmental footprint. Continuous research and development in materials science, design, and control systems are crucial for further advancements in improving the work ratio and the overall efficiency of thermodynamic systems.
Exploring the Connection Between Isentropic Efficiency and Work Ratio
Isentropic efficiency, which describes the deviation of actual processes from ideal isentropic (reversible adiabatic) processes, has a profound effect on the work ratio. In compressors and turbines, the isentropic efficiency quantifies how much of the ideal work is achieved in reality. A lower isentropic efficiency means more work is required to achieve the same pressure change in a compressor (reducing net work output) or less work is produced for a given pressure drop in a turbine (also reducing net work output). Consequently, a lower isentropic efficiency directly leads to a lower work ratio.
For instance, in a Brayton cycle gas turbine, lower isentropic efficiencies of the compressor and turbine significantly reduce the net work output, thereby lowering the work ratio. This highlights the importance of designing efficient turbomachinery to achieve high work ratios and improved cycle efficiency.
Further Analysis of Isentropic Efficiency
Factor | Effect on Isentropic Efficiency | Impact on Work Ratio |
---|---|---|
Blade Design | Improved design increases efficiency | Increases work ratio |
Clearance Losses | Increased clearance reduces efficiency | Decreases work ratio |
Friction Losses | Increased friction reduces efficiency | Decreases work ratio |
Heat Transfer Losses | Heat transfer reduces efficiency | Decreases work ratio |
Manufacturing Tolerances | Imperfect manufacturing reduces efficiency | Decreases work ratio |
FAQ Section
-
Q: What is the ideal work ratio? A: There's no single "ideal" work ratio. It depends entirely on the thermodynamic cycle and its operating conditions. The Carnot cycle represents a theoretical upper limit for efficiency, but real-world cycles always fall short.
-
Q: How does work ratio relate to efficiency? A: Work ratio is directly related to efficiency. A higher work ratio generally implies higher efficiency in converting energy into useful work.
-
Q: Can work ratio be greater than 1? A: Theoretically, it's possible, although unlikely in practical systems. It would require the net work output to exceed the total work input, suggesting some form of energy amplification (e.g., through external energy sources).
-
Q: What units are used for work ratio? A: Work ratio is dimensionless, as it's a ratio of two quantities with the same units (work).
-
Q: How is work ratio used in optimization? A: It serves as a key performance indicator for optimizing cycle parameters, component designs, and operational strategies to maximize energy conversion.
-
Q: What are the limitations of using work ratio? A: Work ratio alone doesn't provide a complete picture of system performance. Other factors, such as cost, environmental impact, and component durability, need to be considered.
Practical Tips
-
Analyze Component Efficiencies: Carefully evaluate the isentropic efficiencies of compressors, turbines, and pumps to identify areas for improvement.
-
Optimize Cycle Parameters: Systematically adjust pressure ratios, temperatures, and other parameters to maximize the net work output.
-
Reduce Friction Losses: Implement designs that minimize friction in moving parts through advanced materials and lubrication techniques.
-
Minimize Heat Losses: Improve insulation and incorporate heat recovery systems to reduce energy loss to the surroundings.
-
Employ Advanced Control Systems: Use sophisticated control systems to maintain optimal operating conditions and maximize efficiency.
-
Consider Alternative Working Fluids: Explore using working fluids with better thermodynamic properties to enhance cycle performance.
-
Utilize Computational Fluid Dynamics (CFD): Use CFD modeling to simulate and optimize component designs for maximum efficiency.
-
Invest in Regular Maintenance: Regular maintenance is essential for minimizing losses and maintaining high work ratios over time.
Final Conclusion
Work ratio stands as a fundamental parameter in thermodynamic analysis, offering invaluable insights into the performance and efficiency of various systems. By understanding its calculation, significance across different cycles, and the factors that influence it, engineers and researchers can develop strategies to improve energy conversion, optimize designs, and create more sustainable and efficient thermodynamic systems. Continued research and innovative engineering solutions will continue to refine our understanding and application of work ratio, driving advancements in energy technology and sustainability.
Latest Posts
Latest Posts
-
What Is The Minimum Payment On A Usaa Credit Card
Mar 31, 2025
-
What Minimum Due Amount Credit Card
Mar 31, 2025
-
Minimum Payment Credit Card
Mar 31, 2025
-
Can You Pay Off A Credit Card With Minimum Payment
Mar 31, 2025
-
What Does Minimum Payment In Credit Card Mean
Mar 31, 2025
Related Post
Thank you for visiting our website which covers about Work Ratio Definition In Thermodynamics . We hope the information provided has been useful to you. Feel free to contact us if you have any questions or need further assistance. See you next time and don't miss to bookmark.